Rainbwo by Ukichiro Nakaya |
“The Blind Girl” is a famous
picture painted by an English painter John Everett Millais.
Beautiful rainbows in the picture make an impression on
the gallery and remain in their remembrance.
This picture
is a view of the English countryside. Two girls, probably are
itinerants, sit side of a road short of a beautiful but empty
meadow. The elder sister is blind. The younger sister cuddles to the
elder and they sit on the grass together. Both sisters
are poorly dressed.
There is a forest over the
meadow, and two rainbows start from the forest to the
dark gray sky. The colors of the rainbows are quite beautiful,
and the rainbows are shining in the painting. The younger sister
faces round the rainbows and says something to the
elder. But the blind girl cannot see the beauty of the rainbows and
retains her posture keeping her accordion on her knee. They
probably are orphans. This painting causes the audience’s pity on
the blind girl of sisters who cannot even see the beauty of the
rainbows.
There is a
small story on these two rainbows. As you know, a big
secondary rainbow appears on the bright and beautiful
primary rainbow. The luminance of the secondary rainbow is weaker
than that of the primary rainbow when two rainbows appear. The
picture caught the brightness of two rainbows. However,
we have to consider another point on the two rainbows. That is the
color order of the rainbows.
Most of the people
believe the colors of a rainbow are the spectral seven colors. And
they remind the word “the seven colors of the rainbow”. The
colors of rainbows are different from the spectral
seven colors in fact, and I will discuss this
issue later. Here, I would like to mention the
color orders of the primary and secondary rainbows. They are
reverse. Red is outside, and purple is inside in the
primary rainbow and purple is outside in the secondary rainbow. All
rainbows show this sequence and have to be so.
However, Millais first
unthinkingly painted rainbows in the same color sequence. This
mistake became a matter, and finally, the second rainbow was
repainted after various discussions. The
picture we see today is the one after repainting.
Junior high
school students learn the color orders of the primary and secondary
rainbows in science class. So, some people may think such a mistake
is ridiculous, and some of them complain about such an
easy mistake on a matter of common knowledge. However, I believe
such people do not know the nature of rainbows exactly. The
complete picture of rainbows is much more complicated than the
conventional explanations found in science textbooks.
Please look at a rainbow
carefully when you find it next time. Many people
believe that the rainbow has seven colors. But only a
few rainbows have seven colors ordering red, orange,
yellow, green, blue, indigo, and purple. Many rainbows show
beautiful red and yellow colors and hardly show blue. On the other
hand, some rainbows show pale red, and some show wide
ranges of yellow and greens and narrow ranges of red and purple. The
seven colors of the rainbow are different from seven
colors of visible spectra. But most of the people fall
into the trap of the word seven colors and idly think
that all rainbows have spectral seven colors. A person who
carefully has observed a rainbow should have questions on the
explanation of rainbows. There is no doubt to have such
questions since the conventional explanations are not enough to
explain the complete picture of rainbows.
Please recall
the image when you showed a brilliant bright rainbow. You might saw
weaker narrow rainbows just inside the primary rainbow. Red and
green lines are eye-catching and doubly or triply appear in this
excess (supernumerary) rainbow. Supernumerary rainbows are
one of the true characters of rainbows since it always
appears concomitantly with a brilliant rainbow.
There are supernumerary rainbows just outer the
secondary rainbow. Though the luminance of these supernumerary
rainbows is weak and difficult to observe, they always
exist. The complete explanation of rainbows should contain the
explanation of these supernumerary rainbows.
Some people also noticed the
difference in the brightness of the sky. The sky
inside the primary rainbow is bright, and the sky outside of the
secondary rainbow is a little bit darker but still bright.
However, the sky between the first and second rainbows is dark.
This difference should be one characteristic
of the rainbow phenomena. If you feel mystery on the beautiful red
and green ring in the sky, the difference of the brightness also
should be a mystery. For a scientific explanation, we cannot say we
understand the phenomena until nothing unexplained remains. Beauty
and eye-catching are problems concerning human feeling
and have no meaning on the importance of the phenomena.
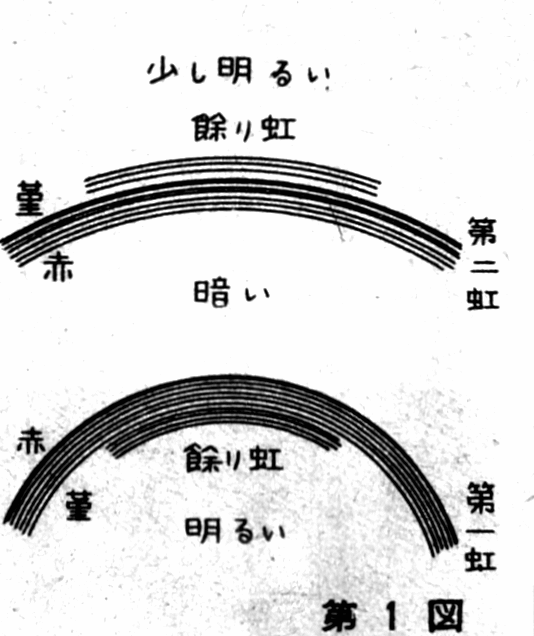
The shape of rainbows is
also a problem. From the conventional explanation of rainbows, the
shape should be perfectly circular, and
the width of rainbows should be the same. However, some rainbows are
ellipsoidal, and some are a little bit
squashed. The width of the rainbows is different among
rainbows. Sometimes the width can be different between parts of a
rainbow. Some people explain these differences from
the difference of conditions of raindrops in the part
of the sky. But he should explain the relationship
between the conditions and the shape of rainbows to make his
explanation perfect. Here, I
will explain the rainbow phenomena step by step. You
finally understand the explanation of rainbow is not so easy as you
have believed from the explanation of science textbooks in a junior
high school.
Let us start the
explanation written in science textbooks. Spectral decomposition
caused by reflection and refraction of light in raindrops acting as
prism creates rainbows. This explanation of the origin
of rainbows is generally correct. Though raindrops
exist all around the sky, not all the sky but only a circular band
of the sky shines. We start to discuss this point first. Then we
will discuss the colorization of a rainbow. If the
sunlight has only a color, then a rainbow becomes a circular band of
the color. For example, you can observe a yellow
rainbow in a laboratory using the monochromatic yellow
light source.
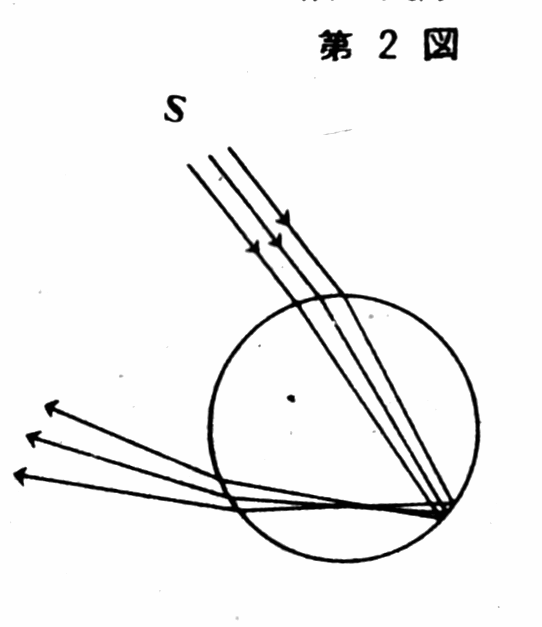
A part of the sunlight
reflected at a surface of a raindrop, and most of the
sunlight enters the droplet refracting at
the surface. The incident light reflects in the droplet and goes out
again, refracting at the surface. Figure 2 shows the simplest case
of the light path. As shown in Fig. 2, the sunlight is a parallel
beam, but the outgoing beam from the droplet is not a parallel beam.
We could not observe a rainbow if there was not a special
characteristic of the light path. If so, reflected
light from every raindrop come to an observer and the
observer see the uniformly bright sky.
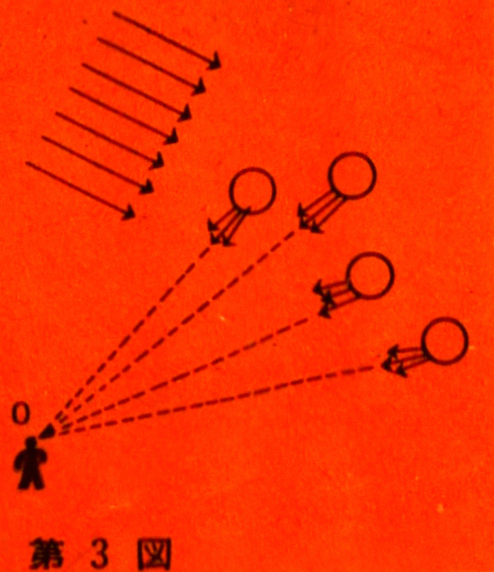
Optical theory shows us that
the light reflected by a raindrop has a special characteristic.
Indeed, the reflected light is not a parallel beam, but the actual
light path is different from that of Fig. 3. I purposely wrote the
incorrect path in Fig. 3. Figure 4 shows the accurate light path
inside and outside of a raindrop. A ray of parallel beam
SP irradiating point P, paths inside the droplet
through the arrows and goes out the droplet to the direction
RT. The other rays incident the droplet at the upper
and lower sides of SP such as 1 and 2, and 3 and 4 exist to the
upper side of the line RT. It seems that rays 3
and 4 exit the lower side of RT if the rays 1 and
2 exit the upper side of RT. However, optical theory shows us
all incident light exit the upper side of RT. P is a singular point
of the raindrop. This singularity comes from the incident angle of
the ray. The incident angle of a sphere is the angle
between the ray and the tangential plane at the incident point.
Output ray goes to the direction RT when the incident
angle is the specific value. Other parallel rays, 1, 2, 3, and
4 incident at any point except for P have
different incident angles and go out upper side of RT. As incident
angle increases, the outgoing rays once become closer to the RT from
the upper side and then back away. The ray density becomes high just
above the line RT. In other words, the outgoing light
becomes strong around this direction.
RT is
such singular direction, and the angle between
the parallel sunlight, and this direction depends on
the refractive index of water and is independent of the size of a
raindrop. The refractive index
does not only depend on materials but also the
wavelength of light. Averaged refractive index of water for white
light is 1.333. Using this value, we obtain 42 degrees as the angle
between SP and RT. The measured angle of the rainbow is
approximately 42 degrees. Now, we can, at least,
explain the origin of the rainbow.
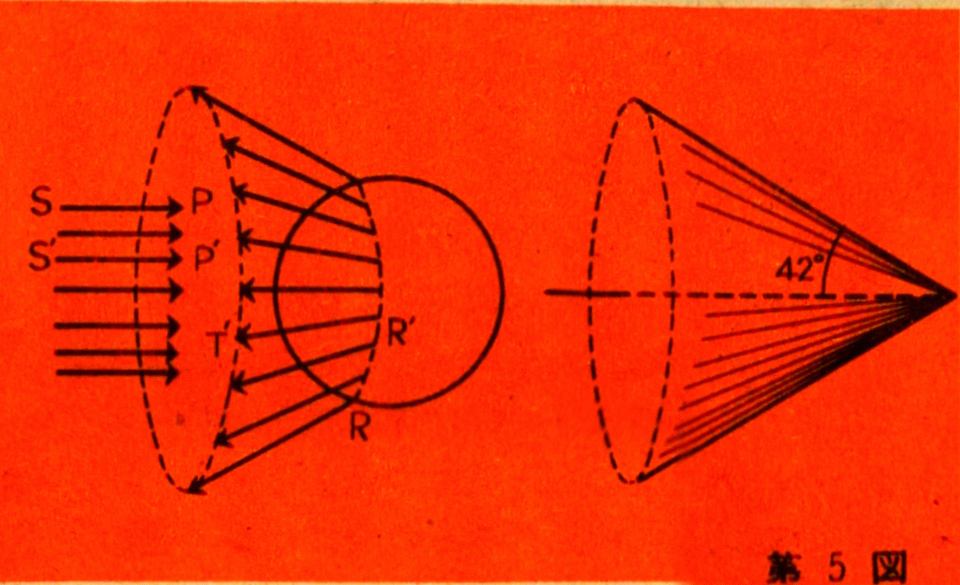
Here, I would like to notice the angle of 42
degrees of the rainbow. In Fig 4, we wrote a raindrop as a circle,
but the real shape of the raindrop is a sphere. Hence, the direction
RT spreads in a 3D space making a circular cone. 42 degrees are the
angle between the axis and face of the circular cone. All
innumerable raindrops in the sky reflect the sunlight in the cone
shape, as shown in Fig. 5. Then the rainbow looks circular for human
eyes like 1, 2, 3, and 4 in Fig. 6. H is the horizontal plane, O is
the observer, and S means the direction of the sun. In this
case, the light comes not only from the raindrops on the arc 1, 2,
3, and 4 but also from all raindrops on the cone. The angle of the
rainbow is the sum of sun height “a” and the height of the rainbow
“b” and the angle is always approximately 42 degrees from
measurements. Hence, we can say the explanation in Fig. 4 is
correct, and we now understand the basic mechanisms of
the rainbow.
The secondary rainbows can
be explained by the similar way. The second rainbow appears outside
the first rainbow, and its angle is approximately 52
degrees. The second rainbow is the rays reflected twice in
raindrops. Figure 7 shows the light path and a particular direction.
In this case, rays 1 and 2, go on the upper, and lower sides of SP
go out the lower side of RT. The light intensity is
strongest when the angle between the parallel incident
ray and the output direction is 52.5 degrees. Also,
no ray returns to the direction smaller than that value.
Optical theory can prove these results. The angle 52.5
degrees coincident with the measured value of the
second rainbow. Now, we can successfully explain the second rainbow.
You may think
that there should be a rainbow created by rays reflected three times
in a raindrop. Not only the third rainbow but also the
fourth and fifth rainbows exist. However, the third and fourth
rainbows appear in the direction of the sun. The strong
sunlight scattered by raindrops hides these rainbows,
and we cannot observe them. The fifth rainbow appears in
approximately the same direction with the secondary rainbow. The
luminance of the fifth rainbow is much weaker than the secondary
rainbow. Hence, we cannot observe the fifth rainbow. The
supernumerary rainbow of the secondary rainbow is different from the
fifth rainbow.
Now I have explained the
basic origin of the primary and secondary rainbows. But I did not
mention the spectral decomposition of the sunlight,
i.e., the origin of colors of rainbows. Now, I will explain this
point.
The
explanation is rather simple. The sunlight is composed
of seven colors such as red and orange etc. Of course,
the phrase “seven colors” means only the typical colors of spectra.
Though real spectral color continuously changes from red to violet,
I will use the phrase seven colors to make explanation simple. The
reflective index of water depends on the colors of light. The
reflective index of the red light is 1.331 and becomes larger toward
the 1.344 of the purple light. The incident white light path through
point P in Fig.8 separate into each color light. The
strongest directions of red and purple light are RT and R’T’,
respectively. The calculation results using indexes for red and
purple light, the output angle of the red and purple light for the
primary rainbow are 42.22 and 40.36 degrees, respectively. The
angles of the red and purple light of the secondary rainbow are
50.24 and 53.36 degrees, respectively. Notice that the magnitude
relation between the red and purple light inverts
between the primary and secondary rainbows. Therefore,
the red and purple parts are outside of the
primary and secondary rainbows, respectively.
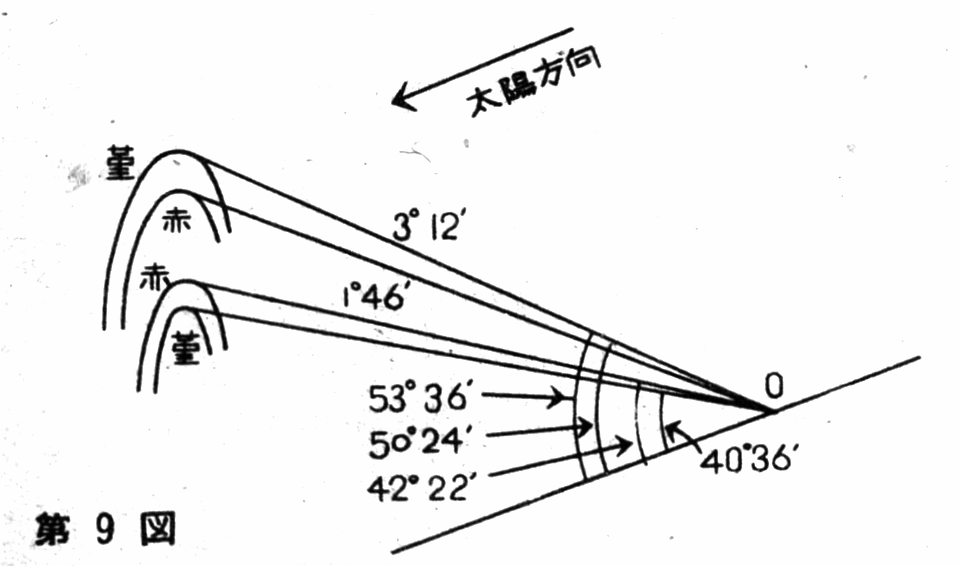
According to
this explanation, the color order of rainbows should be the same.
And all rainbows have spectral seven colors, and their width should
be the same among rainbows. The whole width of the primary rainbow
is 1.46 degree, and that of the second one is 3.12
degrees, as shown in Fig. 9. However, the width of rainbows can be
different, and only a few rainbows show spectral seven colors. These
disagreements clearly show that the conventional explanation written
in the common textbook is not enough.
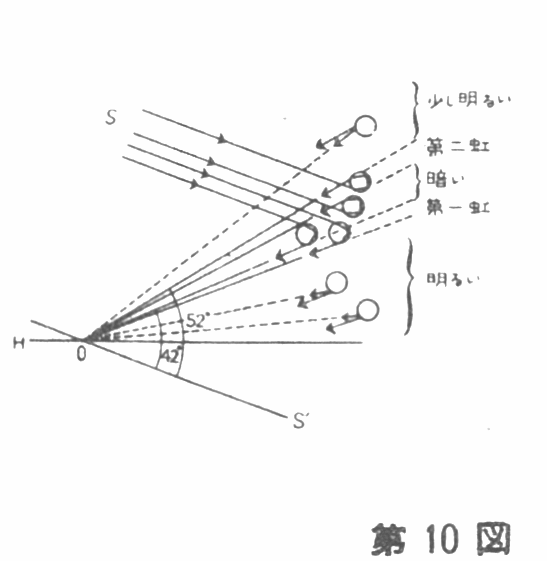
The conventional explanation
cannot explain these features of rainbows, but it can explain the
darkness of the sky between the primary and secondary rainbows. In
Fig. 10, long and short arrows show the strong and weak
outgoing rays. Part of light coming from raindrops inside the
primary rainbow can reach point O. Huge numbers
of raindrops exist inside the primary rainbow and the sky becomes
uniformly bright due to these raindrops. The same is true for
raindrops outside the secondary rainbow. Dotted lines show the light
paths.
No reflected rays from
raindrops between the primary and secondary rainbow can
reach the point O. Rays from raindrops “A” and “B” go
to the direction different from the point O irrespective of the
strength of light. Hence, the sky between the primary
and the secondary rainbows looks darker than the other parts. Light
scattering by the other mechanisms makes the sky of
this area not perfectly dark. The difference in the brightness of
the sky is explained in this way.
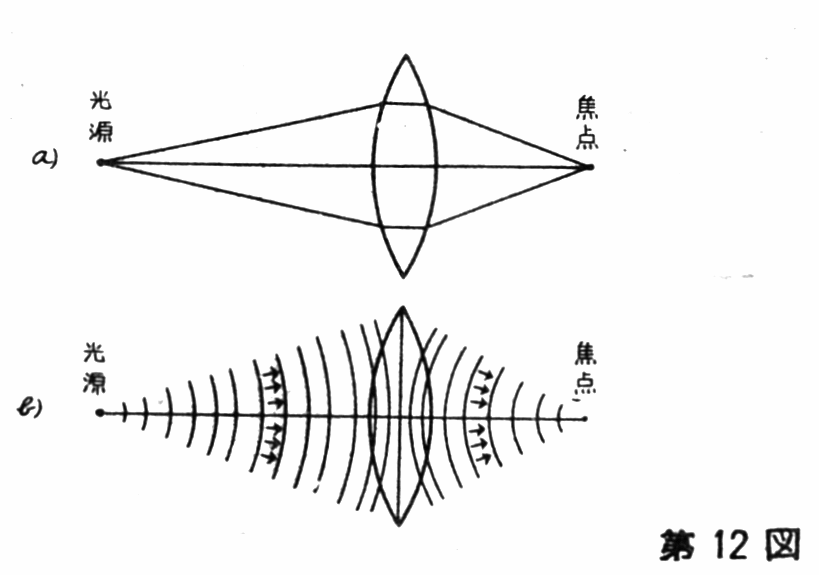
Now, we start the
explanation of the remaining problems, i.e., the width,
color order, and supernumerary rainbows. Here, we have to introduce
a different concept of light. Light beams are
represented by arrows in explanations above. As you know,
light has wave characteristics. Therefore, optical
phenomena should be explained using wave theory in the strict sense.
For example, the collection of light with a lens is typically
illustrated as Fig. 12(a) in the geometrical optics way. However, it
should be illustrated as Fig. 12(b) in the wave optics way. It is
simpler to represent light beam by arrows perpendicular to the
wavefront than by the wavefront themselves. Therefore, the
representation using arrows is commonly used when the
geometrical optics sufficiently explain the optical phenomena, such
as focusing by a lens. Since the wavelength of visible light is as
small as less than 1/1000 millimeter, wave natures of light do not
become a matter for macroscopic objectives. Conversely, we should
take wave nature of light into account, when the size of raindrop
becomes as small as a cloud drop with a diameter of 1/100
millimeter. The explanations of rainbows written above
do not contain the factor of droplet size. We have to
fill in the gaps of explanations by geometrical optics by wave
optics explanations. The appearance of supernumerary
rainbows is well explained by considering the
interference of light when the sunlight is reflected and refracted
in a small raindrop.
Let us reconsider the origin
of the primary rainbow from the wave-optical view. The
wave surfaces are superimposed on the rays
SP and RT in Fig. 4 (Fig. 13). The only wavefront of a plane wave
is added in the figure, in which nothing new exists in
this case. The wave surfaces become curved surface when the
raindrops become so small that the wavelength of light cannot assume
zero compared with the raindrop size. The shapes of the wave
surfaces change from those in Fig. 13 to AOB in Fig. 14. This
change is the consequence of the wave optics
theory. The curvature of AOB depends on the size of a raindrop and
the refractive index of water. The curved surface becomes a
plane surface as the size becomes big enough. The
explanation based on the geometric optical theory is only valid when
the size of raindrops is much bigger than the wavelength of light.
The light beam between AO has convex wave surfaces and diverges.
The light beam between OB has concave wave surfaces
and focuses on point F in Fig. 15. Beyond the point F, this beam
diverges, and the wave surface becomes convex. The
light beam has double wave surfaces (O’A’ and O’B’) far
from the raindrop, as shown in Fig. 15. The double wave surfaces
cause interference.
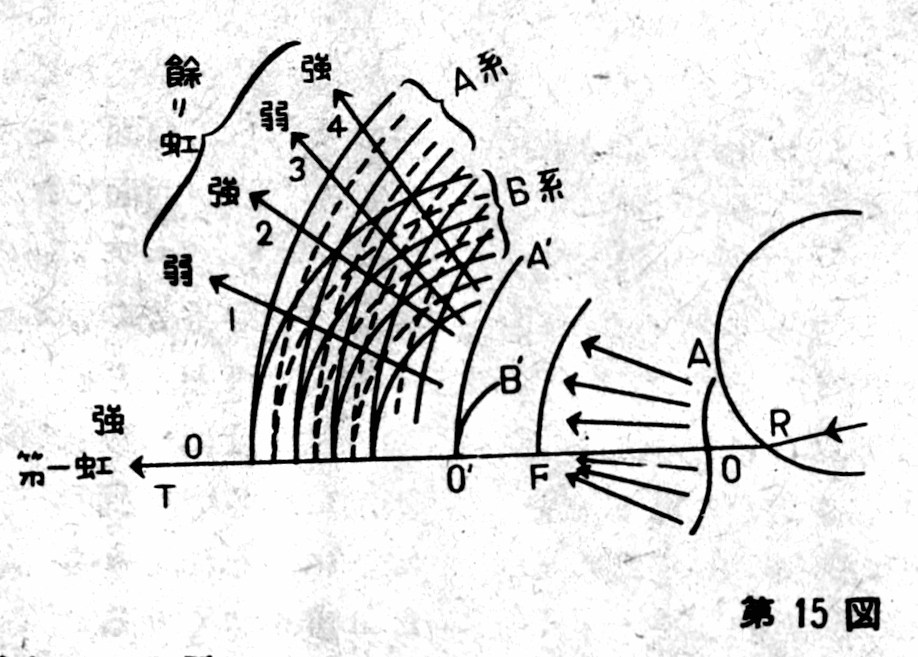
Waves become strong when tops of two waves
overlap and weak when the top and bottom overlap canceling
each other. It is interference of light waves. In Fig.
15, the tops and bottoms of waves are represented by solid
and dashed lines, respectively. The light becomes
intense to the RT direction since tops of A- and B-waves overlap in
this direction. This direction is the front direction of geometrical
optics, and light strength is extremely strong. This
direction corresponds to the corn surface of 42 degrees explained
before. The adding interference happens in the directions of 2 and 4
in Fig. 15 and light intensity becomes strong in these directions.
In the directions of 1 and 3, overlap happens between tops and
bottoms and light waves of this direction become weak.
These interference phenomena create two or three
circular light bands inside the primary rainbow.
These are the true nature of supernumerary rainbows. Usually,
only two or three supernumerary rainbows are observed. Because the
intensity of interference light becomes weak as the angle from the
front direction becomes large. In rare cases, six bands of
supernumerary rainbows are observed.
Above is the outline of the
effect of interference. For the rigorous explanation, we have to
calculate the angles of direction 2 and 4 between that
of direction 0. We also have to check whether the direction 0 agrees
with the angle of 42 degrees explained above. An astronomer
of England, Airy had made this calculation and found that direction
is a little smaller than the value calculated from the geometric
optics. The difference between the geometric and wave
optical view result from the shape of the wave
surface is flat or curved. The wave surfaces become curved planes as
the size of raindrops becomes small. Airy showed the
strongest direction correspond to 42 degrees in large raindrops and
two to three degrees smaller in small raindrops. The drop size
becomes a matter of the wave optics
treatment. Now, we can explain the deviation from the
perfect circular shape. If the raindrop size of a part of the sky is
big and small in the other parts, then the shape of the
rainbow can be warped a little.
Though the above explanation
is not simple, we only deal with monochromatic light at this time.
To explain the spectral future of rainbows, we have to make figures
similar to Fig. 15 for seven colors of spectra and superimpose these
figures. Up to here, most of the characteristics of
rainbows can be explained. Still, we need one more important piece
to explain the whole nature of rainbows.
As I wrote, supernumerary
rainbows always exist and appear inside of the primary rainbow. The
distance, actually the angle distance, between the primary and
supernumerary rainbows causes a new issue. The width of the primary
rainbow is 1.46 degrees. If the angle difference between the primary
and supernumerary rainbows is bigger than this value, then we have
the primary rainbow having bright seven colors and two or three
bands of supernumerary rainbows having faint seven colors inside the
primary rainbow. The angle difference becomes smaller
and bigger when the raindrop size is big and small, respectively.
Therefore, we can observe the perfect primary rainbow involves
supernumerary rainbows inside of it.
When the raindrop is rather
big, then the angle difference between the primary and supernumerary
rainbows becomes smaller than the width of the primary rainbow. In
such case, the reddish part of supernumerary rainbow overlaps with
the blueish part of the primary rainbow. In this type of region,
different color light overlaps and the color reorganization of such
mixed colors become a problem. This problem is different from the
color mixing of paints. We have to know, for example, what kind of
colors we recognize when spectral red and green light mix each
other. The wavelengths of red and green light are different from
each other, and they are unchanged in the mixed light.
The green and red light keep their characteristics in the mixed
light. However, we observed this mixed light quite different from
the green and red light. We feel this mixed light as yellow light.
The simultaneous excitation of red and green light on retina makes
the feeling of yellow. Of course, the ratio between the
green and red light should be appropriate; the mixed
light is practically indistinguishable to the spectral yellow light.
Many of rainbows are such
mixed color ones. The red part of supernumerary rainbow overlaps
with the green part of the primary rainbow makes this part looks
yellow. The first rainbow has a yellow spectral band
outside the green band, and the connection this
original yellow band with the mixed yellow band makes full yellowish
band wide in the primary rainbow. Such a situation happens when the
raindrops are rather large. Such kind of rainbows
is frequently observed after the shower in the
summer. Rainbows tend to bright and
beautiful when the raindrops are big. Please remained
brilliant rainbows after a shower, you will agree that the red and
yellow parts are bright and beautiful and green and blue parts are
faint.
The raindrop
sizes vary among rains and the color order of rainbows can be and
should be different among rainbows.
Now, I have
explained the characteristics of rainbows I showed you at the
beginning of this story. Most people will answer the
reflective and refractive actions when somebody asks them the origin
of rainbows. This answer is not precise as I wrote above, but it is
understandable since they are not scientific scholars.
They may be persons who have better scientific knowledge.
However, the reflective and refractive actions only make rainbows
having seven colors; that is different from most of the
real rainbows with brilliant red and yellow bands. They
have to observe these real rainbows. Assuming that a rainbow
has seven colors without observing real rainbows is a doing go
against a scientific mind.
Natural phenomena are very
complicated and full of mysteries. Most of the phenomena
require more than two pages to explain. Rainbows are a
relatively simple case in the phenomena. But we still need all this
explanation. We have remaining problems on rainbows I
will not discuss here. I believe we can understand the true beauty
of natural phenomena by understanding it one by one.
Some persons
do not try to observe real rainbows, saying that the rainbows are
the spectral actions come from the reflection and refraction by
raindrops. Such persons never understand the beauty of rainbows.
They are who become vision-impaired by science, instead of getting
better vision. Everyone feels pity for the blind girl. Persons who
close their eyes to the natural phenomena are also wretch in other
sense.
Feb 18, 1947
Many websites and popular science books explain the coloring of the rainbow. Though I have not checked all of them even in Japanese, I could not find a better explanation than that written by Ukichiro Nakaya in 1947. He wrote a science essay entitled “Niji” as a contribution to the first number of a science magazine “Niji” for young Japanese. “Niji” is a rainbow in Japanese.
Nakaya died more than 50 years before, and his author’s copyright has vanished in Japan. You can find his essays in Aozora Bunko. Here, I place my translation of “Niji”. I know my English writing is terrible, and I hope someone will revise this translation to better English.
|